Strength and conditioning programmes are essential for improving a horse's overall level of fitness; for reintroducing a horse back into work following an injury, general maintenance or improving fitness for competition. The fundamental importance of correct muscle development is widely understood and considered during the process of strength and conditioning training; however, the cellular and physiological detail is less commonly understood and addressed. Therefore, this review describes the process of muscle growth and looks at how published literature can be applied to maximise the efficiency of conditioning plans to achieve optimal muscle development.
Muscle anatomy
There are three types of muscle: smooth, cardiac and skeletal. Smooth and cardiac muscles are controlled by involuntary neural stimulation. Skeletal muscle is controlled by conscious, voluntary neural stimulation and is responsible for movement (MacIntosh et al, 2006). Therefore, this review looks at the effect of exercise in general, as well as specific, targeted exercises, on skeletal muscle. Skeletal muscle is made up of several subunits – the smallest, sarcomeres, are a contractile unit made up of actin and myosin (Dave et al, 2022). Several sarcomeres are lined in series and in parallel to form myofibrils. Myofibrils are arranged to make muscle fibres, which subsequently become fascicles (Figure 1). The outermost outer layer of skeletal muscle is the epimysium, which is dense, irregular connective tissue (Jennings and Premanandan, 2017).
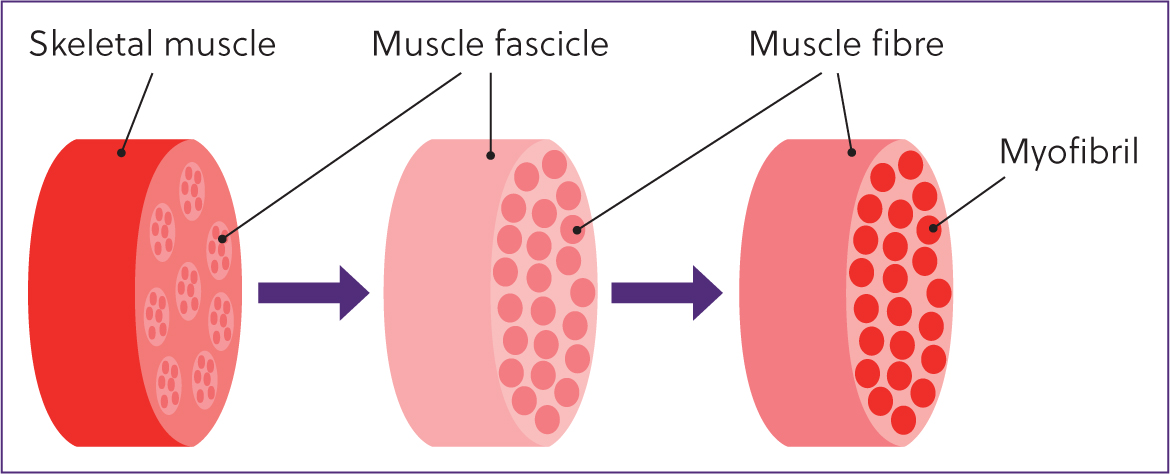
Physiology of hypertrophy
Horses, like many endotherms, stop growing when they reach maturity (Smil, 2020). However, as skeletal muscle is a dynamic tissue, it has the ability to adapt and grow in response to stimuli after maturity, resulting in changes to an individual's stamina, strength and speed (Rivero, 2007; Komi, 2008). Muscle growth can arise either because of hypertrophy or hyperplasia, and can be influenced by various factors including exercise, nutrition, hormones and disease (Frontera and Ochala, 2015). Hypertrophy is an increase in fibre size (Figure 2a), which can occur before and after physiological maturity (Brameld et al, 1998; ST, 2020). Hyperplasia is an increase in the number of muscle fibres (Figure 2b), which occurs predominantly before birth.
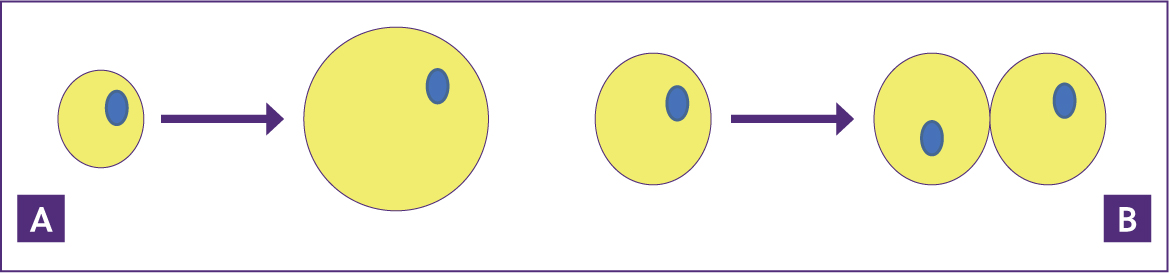
Studies investigating muscle growth in humans and animals have found that hypertrophy is the primary contributing factor of muscle growth after maturity, and hyperplasia alone is only responsible for about 20% of total muscle growth (Sola et al, 1973; Antonia and Gonyea 1993). Any increase in cell number has been thought to be attributed to developing satellite cells (Taylor and Wilkinson, 1986). Exercise can induce hypertrophy by either mechanical tension, metabolic stress or muscle damage (Krzysztofik et al, 2019).
Appropriate muscle mass is a critical component for equine athletic performance (McGivney et al, 2009). Alongside improved performance, increasing muscle mass has also been found to have many health benefits (Figueiredo et al, 2018) including improved cardiovascular function, increased insulin sensitivity, improved inflammatory response and better muscle quality (Hagerman et al, 2000; Ibañez et al, 2005; Brooks et al, 2006; You et al, 2013). Hypertrophy of some equine epaxial muscles has been found to increase stability of the intervertebral joints (De Olivera et al, 2015). Literature reviews and meta-analyses of human studies show that exercise and the subsequent muscular adaptations can modulate metabolism and protect against metabolic disease (Thyfault and Bergouignan, 2020), as well as a positive association between muscular fitness and bone health in adolescents (Smith et al, 2014). These findings primarily relate to humans and therefore it can only be suggested that these benefits might be applicable to horses.
The three types of hypertrophy are myofibrillar, sarcoplasmic and connective tissue hypertrophy, with myofibrillar and sarcoplasmic being the most common (Schoenfeld, 2010). Myofibrillar hypertrophy is a response to an overloading stimulus. The stimulus causes an increase in the size and amounts of the contractile proteins, adding more sarcomeres either in series or in parallel. Therefore, the muscle cross-sectional area increases as a result of muscle damage. Sarcoplasmic hypertrophy is an increase in non-contractile components and fluid, resulting in greater muscle bulk. As the connective tissue in a muscle only accounts for 20% of the total muscle mass, connective tissue hypertrophy contributes the least to overall muscle growth (Komi, 2008).
The physiological contributors involved in skeletal muscle hypertrophy are mechanosensation, muscle remodelling and muscle protein synthesis (McGivney et al, 2009). Muscle protein synthesis is the metabolic process in which amino acids become bound into skeletal muscle, and it is thought to be one of the main contributors to hypertrophy (Witard et al, 2022). Muscle protein synthesis can be stimulated by exercise and correct nutrition, with the anabolic effects lasting up to 24 hours after one exercise session, much longer than nutrition alone which has a shorter, finite effect (~1.5 hours) (Atherton and Smith, 2012). When muscle protein synthesis exceeds muscle protein breakdown, it results in a positive net protein balance and therefore muscle hypertrophy (Damas et al, 2018).
Maintenance and hypertrophy of skeletal muscle also occurs as a result of the proliferation and differentiation of muscle satellite cells. Satellite cells are found adjacent to muscle fibres in a quiescent state, which can be stimulated or inhibited by cytokines and growth factors present during exercise and growth (Reed et al, 2015). When studying isolated satellite cells from four foals, LaVigne et al (2015) found that satellite cell proliferation increased by 28.8% in the presence of insulin-like growth factor-1, and 73% as a result of fibroblast growth factor-2 (P<0.0001). It was also found that fusion was increased 6.2% by interleukin-6 (P≤0.001). Despite the high levels of significance in these findings, the sample size was relatively small which could reduce the reliability of these results.
Exercises and hypertrophy
Several studies have investigated the relation between hypertrophy and exercise in both humans and horses. Damas et al (2018) reviewed evidence regarding the association between skeletal muscle damage and protein synthesis in humans, and the effect of both of these on hypertrophy when resistance training was performed. It was found that the increase in muscle cross-sectional area in the early stages of resistance training (four sessions or fewer) was the result of muscle-damage induced swelling and subsequent repair and remodelling. True hypertrophy was found to occur after 18 sessions, and several weeks of training. It was concluded that resistance training does not have to induce muscle damage to lead to hypertrophy and increased strength.
The findings from this review of human studies indicate that regular training for weeks without necessarily causing muscle damage is the most effective way of achieving true muscular hypertrophy. These data were found from several sources, which increases the reliability of the findings. Further studies would have to be done to investigate if the findings could be repeated within equines, and what species-specific exercises could replicate the effects of human resistance training. If applicable to equines, the conclusions could show that consistent training over several weeks is the best way to achieve true muscle hypertrophy, as the initial increase in muscle size is the result of muscle swelling not hypertrophy.
De Olivera et al (2015) investigated the effect of dynamic mobilisation exercises on the cross-sectional area of the equine multifidus muscle using nine ridden, cross-bred horses. The exercises consisted of cervical flexion, extension and lateral baited stretches, which were done 3 days a week for 3 months. It was found that the cross-sectional area of the multifidus muscle increased by 3.55 cm2, a significant increase compared to the control group. The hypertrophy of this epaxial muscle is responsible for improved stability of the intervertebral joints.
This quantitative measure shows that targeted, dynamic exercises can lead to significant hypertrophy in one muscle. However, this experiment only used nine horses which were randomly allocated to the variable groups. Therefore, the relatively small sample size may reduce the repeatability of the data. Using this data, it could be hypothesised that similar dynamic exercises would also have a positive effect on the growth of any targeted muscle, but further studies would be needed to clarify the reproducibility in other muscles.
Crook et al (2010) undertook a study to investigate the effect of an inclined or declined gradient (-10%, 0 and +10%) on hindlimb muscle activity in horses, by recording the surface electromyo graphic activity of gluteus medius, bicep femoris, vastus lateralis, gastrocnemius and extensor digitorum longus. It was found that a 10% incline on a treadmill increases the mean electromyographic activity of all muscles in walk, and the activity of gluteus medius in trot. However, a 10% decline either reduced the hindlimb electromyographic activity, or there was little activity change in the studied muscles (P<0.05).
These findings could be used to support the potential hypothesis that if there is greater electromyographic activity, there is increased myostimulation, which could lead to increased muscle mass. Despite this study showing either unchanged or reduced electromyographic activity, muscle hypertrophy has been found to still occur regardless of electromyographic activation. In a human study investigating the effect of downhill walking, it was found that in 35 lower limb muscles, there was a 13–32% increase in negative (eccentric) muscle contractions when walking downhill in comparison to a level gradient (Padulo et al, 2013; Hu et al, 2020). Lynn and Morgan (1994) also found that when exercising rats on a 16° decline on a treadmill, there was an increase in sarcomeres in series in the vastus intermedius in comparison to an incline. Despite these studies involving different species and different muscles, it shows that muscle hypertrophy can still be induced by decline work, regardless of the electromyographic activity. However, it could be argued that these subjects are exercising more than usual and therefore any hypertrophy may be induced by the generalised increased workload instead of the gradient. Further studies would be needed to determine the influence of gradient on trained subjects, as well as the repeatability in horses.
One of the most effective stimuli for hypertrophy is mechanical tension. Warneke et al (2022) conducted a meta-analysis of published animal studies to investigate whether stretching could generate sufficient mechanical tension to induce muscle damage and subsequent hypertrophy. This analysis included 89 publications, and following the application of various inclusion and exclusion criteria, the final sample size was 16 controlled studies. Within the included studies, all designs found that long-term stretching caused a significant increase in muscle mass, muscle cross-sectional area, fibre cross-sectional diameter and fibre number. It was concluded that stretching with sufficient intensity and duration induced muscle hypertrophy as a result of micro-traumatisation. Therefore, including a stretching regimen into a horse's routine management may lead to increased muscle mass.
Alongside other factors, the intensity of aerobic exertion also has a connection to muscle hypertrophy. Lindner et al (2013) investigated how the ultrastructural and histochemical features of the equine gluteus medius were affected by different six-week conditioning programmes consisting of varying intensities (blood lactate levels of 1.5 mm, 2.5 mm or 4 mm) and durations (25 or 45 minutes) on 2-year-old sport horses. As well as changes to oxidative capacity by increased mitochondria size, the adaptations included a significant increase in the size of myofibrils. This indicates a significant improvement in muscle aerobic capacity and strength. The exercise intensity which produced blood lactate levels 1.5 mm and 2.5 mm performed for 45 minutes resulted in more significant muscular adaptations, whereas the high intensity, shorter sessions (blood lactate 4 mm) resulted in minimal change. Regardless of the intensity and duration, all muscular and aerobic adaptations returned to their original state after five weeks of inactivity.
The data from this study show that exercise of a low or moderate intensity performed for longer durations (45 minutes) increases muscle stamina and strength more effectively than shorter, high intensity sessions in the gluteus medius. If these findings were shown to be repeatable in other equine muscles, then it would demonstrate that less intense exercise for longer durations would be the most effective way to induce muscle hypertrophy.
Conclusion
As skeletal muscle is a dynamic tissue, it can grow and adapt in response to stimulus; muscle hypertrophy is the primary pathway by which this muscle growth is caused. Muscle hypertrophy leads to several physiological benefits including stabilising equine intervertebral joints, improved cardiovascular function and increased inflammatory response. True hypertrophy has been found to occur after several weeks of training, and can be induced with targeted dynamic exercises. Long-term stretching protocols have been shown to have a continuous, positive effect on stimulating muscle hypertrophy. It has also been found that longer, less aerobically demanding sessions lead to greater muscle growth. If these factors were to be applied wherever relevant, it would potentially increase and maximise muscle growth in horses and therefore achieve optimal athletic performance.
KEY POINTS
- There are three types of muscle: skeletal, cardiac, and smooth. Cardiac and smooth muscle are controlled by involuntary neural stimulation and control processes such as cardiac output and blood vessel dilation, respectively. Skeletal muscle is controlled by conscious stimulation and is responsible for movement; therefore, it has the greatest association with muscle growth.
- Muscle growth can occur in the form of hypertrophy (increase in cell size) or hyperplasia (increase in cell number). The majority of skeletal muscle growth that occurs after maturity is due to hypertrophy, which can be primarily either myofibrillar or sarcoplasmic.
- The physiological contributors to hypertrophy are mechanosensation, muscle remodelling and muscle protein synthesis. Muscle protein synthesis is the main contributor to hypertrophy, and when exercise is performed the duration of anabolic process significantly increases in comparison to nutrition alone.
- In humans and animals, the rate of muscle growth can be increased by using different exercise regimes. It has been found that, in humans, true hypertrophy occurs after 18 sessions and several weeks of training, and within horses the most hypertrophy occurs when the sessions are longer in duration and lower in aerobic intensity. In horses, hypertrophy has also been induced by using targeted mobilisation exercises. The use of different gradients has been found to increase muscle activity in horses, humans, and rodents.